There is an enormous amount of methane (CH4) on earth frozen into a type of ice called methane hydrate. Hydrates can form with almost any gas and consist of a 'cage' of water molecules surrounding the gas. (The term 'clathrate' more generally describes solids consisting of gases are trapped within any kind of cage while hydrate is the specific term for when the cage is made of water molecules). There are CO2 hydrates on Mars, while on Earth most of the hydrates are filled with methane. Most of these are in sediments of the ocean, but some are associated with permafrost soils.
Methane hydrates would seem intuitively to be the most precarious of things. Methane hydrate melts if it gets too warm, and it floats in water. Methane is a powerful greenhouse gas, and it degrades to CO2, another greenhouse gas which accumulates in the atmosphere just as fossil fuel CO2 does. And there is a lot of it, possibly more than the traditional fossil fuel deposits. Conceivably, climate changes could affect these deposits. So what do we know of the disaster-movie potential of the methane hydrates?
Ocean hydrates. Most of the methane hydrate is in sediments of the ocean. Of that, most is what can be called the stratigraphic-type deposits. Organic carbon from plankton is buried over millions of years. Hundreds of meters below the sea floor, bacteria produce methane from the dead plankton. If methane is produced quickly enough, some of it will freeze into methane hydrates. This type of deposit holds thousands of gigatons of carbon as methane [Buffett and Archer, 2004; Milkov, 2004]. For comparison, the most abundant type of traditional fossil fuel is coal, which is typically credited with about 5000 Gton C [Rogner, 1997].
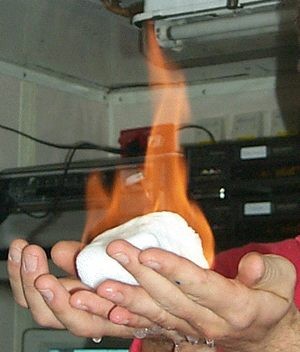
Sometimes the methane moves around in the earth, and collects someplace, forming what are called structural hydrate deposits. The Gulf of Mexico, for example, is basically a leaky oil field [MacDonald et al., 2005]. One implication of gas moving around and pooling like this is that the hydrate concentration can be higher, even to the point of what they call massive deposits, lumps of nearly pure hydrate. The second bottom line is that the hydrate can be found much closer to the sea floor, and even on the sea floor.
Hydrate melts if it gets too warm. The ocean is cold enough in a depth range from say 500 meters down (200 meters in the Arctic). Below the sea floor, the temperature increases with depth, along the geothermal temperature gradient. At some depth it becomes too warm for hydrate, so hydrate melts if it becomes buried deeper than this depth. There is often a layer of bubbles beneath the hydrate stability zone. The bubbles reflect seismic sound waves, and show up clearly in seismic surveys around the world [Buffett, 2000]. Hills and valleys of the bubble layer follow hills and valleys of the sea floor, so this layer is called a bottom-simulating reflector (BSR).

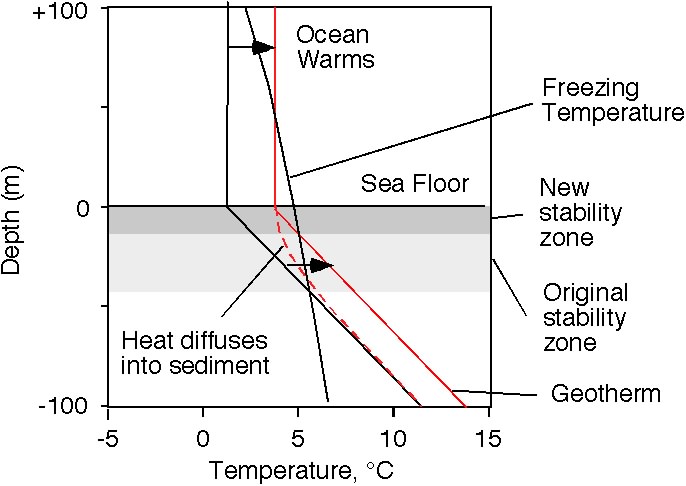
Now let's warm up the water at the top of the sediment column. Ultimately, the new temperature profile will have nearly the same slope as before, the geotherm. The hydrate stability zone will get thinner with an increase in the sediment column temperature.
The important thing to note is that it gets thinner from the bottom, not from the top. Hydrate at the base of the original stability zone finds itself melting.
If the stability zone still exists, it will be shallower in the sediment column than the newly released methane bubbles, and so it could act like a cold trap to prevent the released methane gas from escaping. However, seismic studies often show “wipeout zones” where the BSR is missing, and all of the layered structure of the sediment column above the missing BSR is smoothed out. These are thought to be areas where gas has broken through the structure of the sediment to escape to the ocean [Wood et al., 2002]. One theory is that upward migration of fluid carries with it heat, preventing the methane from freezing as it travels through the nominal stability zone. The sediment surface of the world’s ocean has holes in it called pockmarks [Hill et al., 2004], interpreted to be what these gas explosions look like from the surface.
And there is the possibility of landslides. When hydrate melts and produces bubbles, there is an increase in volume. The idea is that the bubbles might lift the grains off of each other, destabilizing the sediment column. The largest submarine landslide known is off the coast of Norway, called Storegga [Bryn et al., 2005; Mienert et al., 2005]. The slide excavated on average the top 250 meters of sediment over a swath hundreds of kilometers wide, stretching half-way from Norway to Greenland. There have been comparable slides on the Norwegian margin every approximately 100 kyr, synchronous with the glacial cycles [Solheim et al., 2005].
The last one occurred 2-3 kyr years after the stability zone thinned due to increasing water temperature [Mienert et al., 2005], about 8150 years ago. The slide started at a few hundred meters water depth, just off the continental slope, where Mienert calculates the maximum change in HSZ. The Storegga slide area today contains methane clathrate deposits as indicated by a seismic BSR corresponding to the base of the HSZ at 200-300 meters, and pockmarks indicating gas expulsion from the sediment.
However, there is another also apparently plausible hypothesis for Storegga, which doesn't involve hydrates at all. This is the rapid accumulation of glacial sediment shed by the Fennoscandian ice sheet [Bryn et al., 2005]. Rapid sediment loading traps pore water in the sediment column faster than it can be expelled by the increasing sediment load. At some point, the sediment column floats in its own porewater. This mechanism has the capacity to explain why the Norwegian continental margin, of all places in the world, should have landslides synchronous with climate change.
The Storegga slide generated a tsunami in what is now the United Kingdom, but it does not appear to have had any climate connections. It was not a catastrophic amount of methane loss. The volume of sediment moved was about 2500 km3. Assuming 1% hydrate by pore water volume were released on average from the slide volume, you get a methane release of about 0.8 Gton of C. Even if all of the hydrate made it to the atmosphere, it would have had a smaller climate impact than a volcanic eruption (I calculated the methane impact on the radiative budget here).
Actually, the truth be told, the Storegga slide occurred spookily close in time to the 8.2k climate event, but there doesn't appear to be any connection. The 8.2k event was a century-long cool interval, most probably in response to fresh-water release from Glacial Lake Aggasiz to the North Atlantic and was coincident with a ~75 ppbv drop in methane, not a rise.
Methane can leave the sediment in three possible forms: dissolved, bubbles, and hydrate. Dissolved methane is chemically unstable in the oxic water column of the ocean, but it has a lifetime of decades (shorter in high-flux environments) [Valentine et al., 2001], so if the methane is released shallow enough in the ocean, it has a good chance of escaping to the atmosphere. Bubbles of methane are typically only able to rise a few hundred meters before they dissolve. Hydrate floats in water just like regular ice floats in water, carrying methane to the atmosphere much more efficiently than bubbles [Brewer et al., 2002].
For most parts of the ocean, melting of hydrates is a slow process. It takes decades to centuries to warm up the water 1000 meters down in the ocean, and centuries more to diffuse that heat down into the sediment where the base of the stability zone is. The Arctic Ocean may be a special case, because of the shallower stability zone due to the colder water column, and because warming is expected to be more intense in high latitudes.
Permafrost. You've maybe read about permafrost in the paper a lot lately. Permafrost soils are defined as those which remain frozen year-round (actually, the technical definition is a soil which has been frozen for the last two years). There is sometimes a zone near the sediment surface that thaws in the summer. In the permafrost literature, this zone is called the active zone, and it has been observed to be getting larger with time [Sazonova et al., 2004]. Melting of surface soils is one reason why the high latitude Arctic is expected to be a part of the land surface that responds most dramatically to climate change [Bala et al., 2005].
The other reason is that temperature changes are more dramatic in high latitudes than the global average, especially high northern latitudes. There has been a stream of anecdotal reports of the effects of melting permafrosts on the Arctic landscape, tilted buildings and "drunken forests" near Fairbanks, for example [Pearce, 2005; Stockstad, 2004]. Much of the Alaskan oil pipeline is anchored in permafrost soils.
Hydrates are sometimes associated with permafrost deposits, but not too close to the soil surface, because of the requirement for high pressure. The other factor that determines whether you find hydrate is the permeability of the soils. Sometimes freezing, flowing groundwater creates a sealed ice layer in the soil, which can elevate the pressure in the pore space below. Hydrate in a one permafrost core [Dallimore and Collett, 1995] was reported below sealed ice layers. Lakes have been reported to suddenly drain away as some subsurface sealed ice layer is apparently breached.
The grand-daddy of subsurface sealed ice layers is a very large structure in Siberia called the ice complex [Hubberten and Romanovskii, 2001].
The most important means of eroding the ice complex is laterally, by a melt-erosion process called thermokarst erosion [Gavrilov et al., 2003]. The ice layer is exposed to the warming waters of the ocean. As the ice melts, the land collapses, exposing more ice. The northern coast of Siberia has been eroding for thousands of years, but rates are accelerating. Entire islands have disappeared in historical time [Romankevich, 1984]. Concentrations of dissolved methane on the Siberian shelf reached 25 times higher than atmospheric saturation, indicating escape of methane from coastal erosion into the atmosphere [Shakhova et al., 2005]. Total amounts of methane hydrate in permafrost soils are very poorly known, with estimates ranging from 7.5 to 400 Gton C (estimates compiled by [Gornitz and Fung, 1994]).
The Future. The juiciest disaster-movie scenario would be a release of enough methane to significantly change the atmospheric concentration, on a time scale that is fast compared with the lifetime of methane. This would generate a spike in methane concentration. For a scale of how much would be a large methane release, the amount of methane that would be required to equal the radiative forcing of doubled CO2 would be about ten times the present methane concentration. That would be disaster movie. Or, the difference between the worst case IPCC scenario and the best conceivable 'alternative scenario' by 2050 is only about 1 W/m2 mean radiative